25: SELF-SERVICE (Self-X, Automation, Self-Organization, Self-Healing etc): (A) Make an object (or system) to serve itself and (B) Carry out supplementary operations (like repair or correct or organize etc), (C) use waste resources (available at no or low additional expense) – material or energy or time.C
SYNONYMS : Self-X, Automation, Self-Organization, Self-Healing, Self-Sealing, Self-Correcting…
EXAMPLE: Self-Balancing Wheel, Self-Cleaning Filters, Halogen Lamps (Regenerating Filament During Use), Biofuel/Fertilizer, Dynamo, Organic Fertilizers, Using Heat, Data Driven Software Algorithm, Self-Testing Software, Combined Heat and Power (CHP) Systems, Automated Teller Machine (ATM), (Food) Ordering or Vending (Dispensing) Kiosks or Machines, Mobile Application (Banking/Investing), (Airport/Hotel) Self-Service Check-in Kiosks, Self-Service Laundary , Self-Healing (Medidation, Biomimetics), Auto-Correction (Spelling, Grammer etc), Self-Healing (like wrinkle free clothes) Synthetic Material or Polymers (Self-Sealing or Restore After Damages) .
ACB:
Benjamin Franklin attended school in Boston for only two years, cut wicks and melted tallow in his father’s candle shop, and at seventeen ran away to Philadelphia. As a boy, Benjamin Franklin taught himself algebra, geometry, navigation, grammar, logic, French, German, Italian, Spanish and Latin. As an adult, he founded the Pennsylvania Gazette, published Poor Richard’s Almanac, proved that lightening is electricity, invented bifocal lenses, founded the University of Pennsylvania, served as minister to France and signed the Declaration of Independence, and the United States Constitution.
The inventive principle encourages inventive thinking in designing systems that not only fulfill their primary functions but also actively manage and optimize their own operation. By reducing the dependency on external control and human involvement, systems become more efficient, reliable, and adaptable to varying conditions. The principle can be employed in various contexts, from technological innovations to process improvements, to enhance the autonomy and effectiveness of systems. “Self-Service” is associated with the idea of designing systems or processes that enable users or components to perform functions autonomously without direct external intervention. The concept of self-service aims to empower the system or its components to fulfill certain tasks independently, reducing the need for external control or manual operation. This principle is often applied in various fields, such as automation, user interfaces, and process optimization. The goal is to design systems that can operate with minimal human intervention, providing services or performing functions in a self-directed manner.
Designing an object to service itself and perform supplementary and repair operations involves creating a system that can autonomously maintain and repair itself as needed. By implementing these features, the object becomes more resilient, reliable, and self-sustaining, reducing the need for external intervention and ensuring continuous operation even in challenging environments. This concept of self-servicing objects holds promise for various applications, including robotics, transportation, infrastructure, and consumer electronics. This concept aligns with the principles of self-healing and self-maintenance in engineering and technology. Here’s how it could work:
Self-Diagnosis: The object is equipped with sensors and diagnostic tools to continuously monitor its own condition and performance. It can detect abnormalities, faults, or wear and tear in its components. Self-Repair Mechanisms: Upon detecting issues, the object activates self-repair mechanisms to address the problems. This could involve internal systems such as 3D printers for manufacturing replacement parts, robotic arms for assembly, or nanotechnology for repairing damaged components at the molecular level. Supplementary Operations: In addition to basic functions, the object is designed to perform supplementary operations that enhance its functionality or efficiency. For example, a self-driving car could autonomously schedule and perform maintenance tasks such as tire rotation, oil changes, or software updates. Remote Monitoring and Control: The object is connected to a centralized control system that enables remote monitoring and control. This allows for real-time tracking of the object’s condition and performance, as well as the ability to initiate repair or maintenance actions remotely when necessary. Adaptive Learning: The object incorporates machine learning algorithms to adapt and improve its self-maintenance and repair capabilities over time. It learns from past experiences and feedback to optimize its performance and anticipate future maintenance needs. Modular Design: The object is designed with modular components that can be easily replaced or upgraded as needed. This facilitates repair and maintenance operations by allowing for quick and efficient component replacement without requiring specialized tools or extensive downtime.
Making use of waste materials and energy involves implementing strategies to repurpose, recycle, or harness resources that would otherwise be discarded or wasted. By making use of waste materials and energy, organizations can reduce their environmental footprint, lower operating costs, and contribute to a more sustainable and resource-efficient future. These practices not only benefit the environment but also create economic opportunities and support the transition to a circular economy. This approach promotes sustainability, reduces environmental impact, and maximizes resource efficiency. Here are some ways to achieve this:
Recycling and Upcycling: Implement recycling programs to collect and process waste materials such as paper, plastic, glass, and metals. These materials can be transformed into new products through recycling or upcycling processes, reducing the need for virgin resources and minimizing waste. Waste-to-Energy Conversion: Utilize waste-to-energy technologies to convert organic waste, biomass, or municipal solid waste into heat, electricity, or biofuels. Technologies such as anaerobic digestion, incineration, and gasification can generate energy from organic waste streams while reducing landfill volumes and greenhouse gas emissions. Circular Economy Practices: Adopt circular economy principles to design products, processes, and systems that minimize waste and maximize resource recovery. This involves designing products for durability, reusability, and recyclability, as well as implementing closed-loop recycling systems to recover and reintegrate materials into the production cycle. Energy Recovery from Industrial Processes: Implement energy recovery systems in industrial facilities to capture and reuse waste heat or kinetic energy generated during manufacturing processes. Heat recovery technologies such as heat exchangers, cogeneration systems, and waste heat boilers can recover thermal energy for space heating, water heating, or power generation. Biogas Production from Organic Waste: Utilize anaerobic digestion systems to convert organic waste materials such as food scraps, agricultural residues, and wastewater sludge into biogas, a renewable energy source composed primarily of methane. Biogas can be used for heating, electricity generation, or vehicle fuel, displacing fossil fuels and reducing greenhouse gas emissions. Renewable Energy Integration: Integrate renewable energy sources such as solar, wind, and hydroelectric power into waste management and resource recovery processes. Solar panels, wind turbines, and micro-hydro systems can generate clean energy to power waste treatment facilities, recycling plants, and other waste management infrastructure.
Making use of available resources in an external or surrounding environment involves leveraging existing resources to achieve specific goals or outcomes. This approach is common in various fields, including engineering, ecology, and urban planning. By making use of available resources in the external environment, organizations can optimize resource utilization, minimize environmental impact, and promote sustainable development. This approach requires careful planning, collaboration, and innovation to balance economic, social, and environmental priorities effectively. Here are some ways to utilize different types of resources:
Information: Gather and analyze information from external sources such as databases, research studies, and expert knowledge to inform decision-making and problem-solving. Utilize data analytics tools and techniques to extract insights and patterns from large datasets, enabling evidence-based decision-making and optimizing processes. Energy: Harness renewable energy sources such as solar, wind, and hydroelectric power to generate electricity and heat. Install solar panels, wind turbines, or hydroelectric generators to capture renewable energy resources and reduce reliance on fossil fuels. Implement energy-efficient technologies and practices to minimize energy consumption and maximize efficiency. Substances: Utilize natural resources and raw materials available in the surrounding environment for manufacturing, construction, or production processes. Recycle and repurpose waste materials such as metals, plastics, and organic matter to minimize resource depletion and reduce environmental impact. Implement circular economy principles to promote resource recovery and closed-loop recycling systems. Space: Optimize the use of physical space in urban, rural, or industrial environments to maximize efficiency and functionality. Design compact and flexible layouts for buildings, infrastructure, and transportation networks to minimize land use and maximize productivity. Implement green space initiatives to enhance biodiversity, improve air quality, and provide recreational opportunities in urban areas. Ecological Resources: Preserve and protect natural ecosystems and biodiversity by conserving habitats, reducing pollution, and promoting sustainable land use practices. Implement ecosystem-based management strategies to restore degraded landscapes, enhance soil fertility, and mitigate climate change impacts. Utilize ecosystem services such as pollination, water filtration, and carbon sequestration to support human well-being and ecosystem health.
Making operations sustainable involves implementing strategies to minimize harm to both the system itself and its external environment. By removing or reducing harm to both the system itself and its external environment, organizations can promote sustainability, minimize risk, and contribute to the well-being of ecosystems and communities. This approach requires a holistic and proactive approach to managing environmental impacts and prioritizing the long-term health and resilience of both the system and its surrounding environment. Here’s how this can be achieved:
Reduce Resource Consumption: Implement measures to reduce resource consumption within the system, such as energy, water, and raw materials. This can involve optimizing processes, upgrading equipment to more energy-efficient models, and implementing recycling and reuse programs to minimize waste. Pollution Prevention: Implement pollution prevention measures to reduce the release of harmful emissions, pollutants, and waste into the environment. This can involve implementing pollution control technologies, improving waste management practices, and adhering to regulatory standards for air and water quality. Environmental Impact Assessment: Conduct environmental impact assessments to identify potential risks and impacts associated with operations. This involves evaluating the environmental, social, and economic consequences of activities and identifying opportunities for improvement. Sustainable Sourcing: Source materials, products, and services from suppliers that prioritize sustainability and environmental stewardship. This can involve sourcing renewable or recycled materials, supporting local suppliers, and ensuring adherence to ethical labor practices throughout the supply chain. Ecosystem Protection: Take measures to protect and preserve natural ecosystems and biodiversity in the surrounding environment. This can involve implementing conservation measures, restoring degraded habitats, and minimizing habitat destruction and fragmentation. Community Engagement: Engage with local communities and stakeholders to understand their concerns and priorities regarding environmental sustainability. This can involve consulting with communities, providing transparency about operations, and collaborating on initiatives to address shared environmental challenges. Continuous Improvement: Implement a culture of continuous improvement to identify opportunities for reducing harm and enhancing sustainability over time. This involves monitoring performance, setting targets and goals for improvement, and regularly reviewing and updating practices and procedures.
This emphasizes the importance of self-care, particularly in relation to productivity. Just like humans need to prioritize their well-being to maintain optimal performance, technical systems too. Taking breaks, including engaging in activities like exercise, is highlighted as crucial for releasing pressure and tension. The author suggests that incorporating self-care practices into one’s routine leads to clearer thinking and higher-quality work output. The machines too need to be self-aware of its state of operation and health and plan self-care through automated breakes and preventive care. Preventive mainteance is also part of continuity of useful action, periodic action and prior counteraction, how in case of this principle, this implies that it is being carried out by the system itself and it does not lead itself to state of breakdown.
Preventive maintenance is a critical component of ensuring the reliability, longevity, and efficiency of equipment, machinery, and systems. It involves proactively identifying and addressing potential issues before they escalate into costly breakdowns or failures. By integrating preventive maintenance practices into the overall approach, organizations can minimize downtime, reduce maintenance costs, and optimize asset performance and reliability. Preventive maintenance complements predictive, proactive, and reactive maintenance strategies, forming a comprehensive maintenance strategy that ensures the long-term success and sustainability of operations. Here’s how preventive maintenance can be integrated into the approach outlined:
Predictive Maintenance Techniques: Utilize predictive maintenance techniques, such as condition monitoring and predictive analytics, to anticipate equipment failures and identify maintenance needs in advance. By analyzing real-time data and performance metrics, organizations can detect early warning signs of potential issues and take proactive measures to address them. Scheduled Inspections and Maintenance: Implement scheduled inspections and maintenance routines to regularly assess the condition of equipment and perform necessary repairs or adjustments. Develop maintenance schedules based on manufacturer recommendations, industry best practices, and operational requirements to ensure consistent performance and reliability. Equipment Health Monitoring Systems: Install equipment health monitoring systems to continuously monitor the condition of critical assets and detect deviations from normal operating parameters. These systems can provide early warnings of potential failures, allowing maintenance teams to intervene before problems escalate. Reliability-centered Maintenance (RCM): Adopt a reliability-centered maintenance approach to prioritize maintenance activities based on the criticality and impact of assets on overall operations. Identify the most critical components and systems that require regular monitoring and maintenance to prevent failures and minimize downtime. Proactive Replacement of Components: Proactively replace or refurbish components and parts that are prone to wear and degradation before they reach the end of their useful life. By replacing components on a scheduled basis, organizations can prevent unexpected failures and extend the lifespan of equipment. Training and Skills Development: Invest in training and skills development programs for maintenance personnel to ensure they have the knowledge and expertise to perform preventive maintenance tasks effectively. Provide training on equipment operation, maintenance procedures, and troubleshooting techniques to empower maintenance teams to identify and address issues proactively. Documentation and Record-keeping: Maintain comprehensive documentation and records of maintenance activities, including inspection reports, work orders, and equipment histories. This information provides valuable insights into equipment performance, maintenance trends, and historical issues, facilitating data-driven decision-making and continuous improvement.
To leverage prediction, metacognition, and generative intelligence for self-learning and self-assessment driven introduction of new behaviors, capabilities, and actions, an integrated approach is needed. By integrating prediction, metacognition, and generative intelligence into self-learning and self-assessment processes, individuals and organizations can enhance their agility, adaptability, and resilience in an ever-changing environment. This integrated approach enables continuous learning, improvement, and innovation, driving sustainable growth and success. Here’s how it can be implemented:
Prediction: Utilize predictive analytics and machine learning algorithms to anticipate future trends, outcomes, and opportunities. By analyzing historical data and patterns, the system can make predictions about potential scenarios and their likelihood of occurrence. These predictions serve as a basis for informed decision-making and proactive planning. Metacognition: Incorporate metacognitive strategies to enhance self-awareness, self-reflection, and self-regulation. Encourage individuals to reflect on their thoughts, feelings, and behaviors, as well as their learning processes and strategies. By fostering metacognitive skills, individuals can better understand their strengths and weaknesses, identify areas for improvement, and adapt their learning strategies accordingly. Generative Intelligence: Harness generative intelligence to explore creative solutions and novel approaches to problem-solving. Encourage experimentation, exploration, and innovation to generate new ideas and insights. By promoting divergent thinking and creative problem-solving, individuals can uncover new opportunities and challenge conventional assumptions. Self-Learning Algorithms: Develop self-learning algorithms that adapt and evolve based on feedback and experience. These algorithms continuously analyze performance data, identify patterns and trends, and adjust their behavior accordingly. By leveraging machine learning and artificial intelligence techniques, the system can autonomously improve its performance and effectiveness over time. Self-Assessment Tools: Provide self-assessment tools and mechanisms for individuals to evaluate their own performance, progress, and development. These tools may include quizzes, surveys, self-assessment rubrics, or reflective prompts. By engaging in self-assessment activities, individuals can identify areas of strength and weakness, set learning goals, and track their progress over time. Feedback Loops: Establish feedback loops to facilitate ongoing reflection, evaluation, and adjustment. Encourage individuals to seek feedback from peers, mentors, and stakeholders, as well as from the system itself. By soliciting feedback from multiple sources, individuals can gain different perspectives and insights that inform their self-learning and self-assessment efforts. Continuous Improvement Culture: Foster a culture of continuous improvement and lifelong learning within the organization. Encourage individuals to embrace a growth mindset, where challenges are viewed as opportunities for learning and development. By promoting a culture of curiosity, experimentation, and adaptation, the organization can drive innovation and excellence.
At an abstract level, this inventive principle represents the concept of designing systems, processes, or interfaces in a way that enables them to operate autonomously, perform functions without constant external control, and empower users or components to accomplish tasks independently. It encourages creative solutions that enable systems to operate with a degree of self-sufficiency, contributing to increased effectiveness and adaptability. The application of this principle can vary across different domains, from technology and engineering to user experience design and organizational processes.It encourages the development of systems that have a degree of self-direction, reducing the need for manual intervention and enhancing efficiency. Designing mechanisms, technologies or systems that can automate repetitive or routine tasks or processes, reducing the need for manual intervention. Designing interfaces, algorithms (unsupervised or re-inforcement learning) or systems that can function independently, make decisions, and execute tasks without continuous external guidance. Enabling systems to operate autonomously, making decisions and executing functions based on predefined rules or conditions. Implementing sensors and feedback mechanisms to allow the system to perceive its environment and adjust its actions accordingly. Integrating smart technologies, artificial intelligence, or machine learning to enhance the system’s ability to learn and adapt to changing conditions. Streamlining processes to minimize the need for manual steps, allowing the system to operate seamlessly and efficiently. Streamlining operations to minimize the need for external control, making the system more self-sufficient and effective. Incorporating features that enable systems to diagnose issues, perform maintenance, or address problems without external intervention. Designing intuitive and user-friendly interfaces that facilitate self-service options for end-users. Providing users or components with the capability to perform tasks without relying on external assistance.
The inventive principle of “Self-Service” can be applied to resolve various business and technical contradictions by introducing autonomy, efficiency, and user empowerment. Implementing self-service kiosks or automated systems to allow customers to perform transactions independently, reducing the need for manual labor and increasing operational efficiency. Introducing self-service customer support options, such as AI-driven chatbots or interactive voice response (IVR) systems, to address common queries and issues without immediate human intervention. Implementing self-service data entry interfaces with validation checks and automation to minimize manual errors and improve data accuracy. Developing user-friendly interfaces and self-service training modules to empower users to acquire necessary skills independently, reducing the need for extensive training programs. Creating self-service portals or knowledge bases that allow users to access information, resources, and support without relying on assistance from experts or support staff.
Implementing self-service check-in kiosks, mobile apps, or online platforms to reduce waiting times and enhance the overall customer experience in industries such as transportation and hospitality. Integrating sensors and predictive maintenance algorithms to enable self-service diagnostics and proactive maintenance, reducing the need for frequent manual inspections. Empowering employees with self-service tools, automation, and decision support systems to enable them to perform tasks more autonomously and increase overall productivity. Implementing self-adaptive manufacturing systems that can adjust production parameters based on real-time data and changing conditions, reducing the need for manual adjustments. Introducing smart and self-regulating systems that optimize energy usage based on demand and environmental conditions, contributing to energy efficiency and cost savings. Designing intuitive and self-explanatory user interfaces that enable users to interact with systems without the need for extensive training or guidance.
Self-cleaning windows or glass, often referred to as “self-cleaning glass,” incorporate technologies that help keep the glass surface free from dirt, dust, and other contaminants. Two common technologies used for self-cleaning glass are: Hydrophobic Coatings: Hydrophobic coatings make the glass surface water-repellent. These coatings reduce adhesion of water droplets and encourage them to form beads that roll off the glass surface, carrying away dirt and contaminants. Titanium dioxide (TiO2) is commonly used in hydrophobic coatings. When exposed to ultraviolet (UV) light, TiO2 becomes photocatalytic, breaking down organic matter on the glass surface. Photocatalytic Coatings: Photocatalytic coatings, often combined with hydrophobic elements, use the power of sunlight to trigger a chemical reaction on the glass surface. This reaction helps break down and decompose organic pollutants. Titanium dioxide is a key component in many photocatalytic coatings. When exposed to sunlight, TiO2 generates reactive oxygen species, which interact with organic materials and contribute to the self-cleaning effect.
Self-cleaning glass reduces the frequency of manual cleaning, lowering maintenance costs for buildings. Since self-cleaning glass relies on natural processes and sunlight, it can contribute to a more environmentally friendly approach to building maintenance. Clear and clean glass enhances the aesthetics of buildings, providing better views and allowing more natural light to enter. While self-cleaning glass can reduce the need for manual cleaning, it may not eliminate the need entirely, especially in areas with heavy pollution or where the glass is not exposed to sunlight regularly. The effectiveness of self-cleaning glass can depend on environmental factors, such as the amount of sunlight the glass receives and the types of contaminants in the area. Several companies offer self-cleaning glass solutions, and the technology continues to evolve to enhance performance and durability.
Self-service systems empower users to perform tasks or access information independently without requiring direct assistance from a service provider or staff. These systems are designed to enhance efficiency, convenience, and user experience. Users can perform various banking transactions such as withdrawing cash, depositing money, transferring funds, and checking account balances without visiting a bank teller. Shoppers can scan and pay for their purchases without the assistance of a cashier. These kiosks often allow customers to bag their items and complete the transaction independently. Users can manage their accounts, transfer funds, pay bills, and conduct financial transactions using online banking websites or mobile apps without visiting a physical bank branch. Passengers can check in, print boarding passes, and select seats without assistance from airline staff, enhancing the check-in process at airports. Guests can check in, obtain room keys, and manage reservations using self-service kiosks, reducing the need for front desk assistance. AI-driven chatbots or interactive voice response (IVR) systems allow users to get information, resolve issues, or perform specific tasks without speaking to a live customer service representative.
Library patrons can borrow and return books using self-checkout stations, reducing the need for librarian assistance during routine transactions. Users can purchase tickets for public transportation, concerts, movies, or events using automated vending machines without queuing at a ticket counter. Customers can refuel their vehicles, pay for gas, and access convenience store items at self-service gas stations without the assistance of an attendant. Employees can manage leave requests, update personal information, and access HR-related services through self-service portals without direct HR involvement. Users can book flights, hotels, rental cars, or other services through online platforms without the need for travel agents. Users can wash and dry their clothes using self-service laundry facilities, where they operate the machines and complete the entire laundry process independently.
Wrinkle-free clothes, also known as non-iron or easy-care garments, are designed to resist wrinkles and maintain a smoother appearance, even after washing and drying. This is achieved through various technologies and treatments applied to the fabric during manufacturing. Fabrics are treated with chemicals, such as formaldehyde-based resins or other wrinkle-resistant agents, during the manufacturing process.These chemicals cross-link with the fibers in the fabric, creating a more stable structure that resists wrinkling. Some wrinkle-free fabrics undergo mechanical processes, such as heat-setting or calendaring, to set the fibers in a particular orientation. This mechanical treatment helps the fabric retain its smooth appearance even after washing and drying.
Wrinkle-free clothes require less ironing or steaming, saving time and effort in garment care. The fabric’s resistance to wrinkles ensures that the garment maintains a crisp and neat look throughout the day. There can be a trade-off between comfort and wrinkle resistance. The chemicals used in some wrinkle-free treatments may affect the breathability or feel of the fabric. Some of the chemicals used in wrinkle-free treatments, such as formaldehyde-based resins, have raised environmental and health concerns. The contradiction involves achieving wrinkle resistance while minimizing the environmental impact and potential health risks associated with certain chemicals. The processes and treatments that make fabrics wrinkle-resistant may impact the overall durability and longevity of the garment. Addressing this contradiction involves finding ways to enhance the durability of wrinkle-free fabrics without compromising their resistance to wrinkles. While wrinkle-free clothes offer convenience and a polished appearance, addressing the associated contradictions is essential to ensure that the garments meet comfort, environmental, and durability standards. Advances in textile technology continue to explore new methods for achieving wrinkle resistance while minimizing potential drawbacks.
Touch screens work through a technology called capacitive sensing. Capacitive touch screens are coated with a transparent conductive material, typically indium tin oxide (ITO). When a finger touches the screen, it creates a capacitive coupling between the finger and the screen. The human body is conductive. The touch screen constantly emits an electrostatic field across the surface. When a finger comes into contact with the screen, it disrupts the electrostatic field locally. The touch screen is divided into a grid of rows and columns of sensors. The disruption in the electrostatic field is detected by the sensors, pinpointing the exact location of the touch. The touch screen controller processes the input data from the sensors and determines the touch location. The device’s software then interprets this information and performs the corresponding action.
Using fingers for touch input is natural and intuitive, as it mimics the way we interact with physical objects. It eliminates the need for additional tools like a stylus, making the interface more user-friendly. Fingers enable multi-touch gestures, allowing users to perform actions like zooming or rotating with multiple fingers simultaneously. Fingers offer a user-friendly interface, but they may lack the precision of a stylus. This contradiction is often addressed by optimizing touch sensitivity and implementing features like pinch-to-zoom for finer control. Using fingers simplifies interaction but can pose challenges for intricate tasks. Touch screens address this by incorporating gestures and multi-touch functionality to enhance the range of actions.
Finger touch mirrors natural interactions and gestures, reducing the learning curve for users. Fingers are always available, eliminating the need to carry and manage an additional stylus or external device. Fingers allow for simultaneous multi-touch gestures, providing a more versatile and dynamic user experience. While finger touch has become the standard for most touch screens, styluses and external devices are still used in specific applications where precision is crucial, such as graphic design or note-taking on tablets.
Innovative systems can turn outputs that might traditionally be considered waste into valuable resources, contributing to environmental sustainability and resource efficiency. They often embody principles of circular economy and cosed loop systems. In closed-loop recycling systems, materials such as plastics, metals, or paper are collected, processed, and reused to manufacture new products. The output (waste) from the system becomes the input for the next cycle of production. Anaerobic digestion systems process organic waste (e.g., food scraps, agricultural residues) to produce biogas and nutrient-rich digestate. The biogas can be used for energy generation, and the digestate can serve as fertilizer for crops. Wastewater treatment plants purify sewage water to meet environmental standards. Treated water can be reused for irrigation, industrial processes, or even potable water supply, minimizing the discharge of pollutants into the environment. Waste-to-energy facilities incinerate municipal solid waste to generate electricity. The heat produced during incineration is used to produce steam that drives turbines, converting waste into a valuable energy resource.
Closed-loop food systems, often seen in sustainable agriculture, involve using food waste as compost or feed for animals. This closed-loop approach minimizes food waste and enhances the overall efficiency of the agricultural system. In circular fashion systems, textile waste is collected, processed, and used as raw material for creating new garments. This approach reduces the environmental impact of the fashion industry by extending the life cycle of textiles. Electronics manufacturers implement closed-loop systems where end-of-life electronic products are collected, disassembled, and the components are reused to manufacture new electronic devices, minimizing electronic waste.
Some packaging materials are designed to be biodegradable or compostable. After use, these materials break down into organic matter, enriching the soil and reducing the environmental impact compared to traditional packaging. Agricultural systems that focus on regenerative practices, such as cover cropping and crop rotation, contribute to soil health and fertility. Organic matter from crop residues becomes a beneficial input for future crops. Industrial processes that treat and recycle water within a closed-loop system reduce water consumption and minimize the discharge of wastewater into the environment. Upcycling involves repurposing discarded materials or products to create new, higher-value items. For example, turning old denim jeans into fashionable accessories. Closed-loop systems for plastic recycling involve collecting used plastic products, processing them into recycled plastic, and using that material to manufacture new plastic products.
Memory biases, such as misattribution, typically involve cognitive processes and perceptions that are influenced by various factors such as past experiences, beliefs, and social influences. While taking proactive steps to manage one’s memory, such as using mnemonic devices or organizational strategies, may help reduce the occurrence of memory biases to some extent, it’s challenging to completely eliminate them through prior action alone. Encouraging verification and validation of information through multiple sources can help mitigate the risk of misattribution by providing additional context and corroboration. Metacognition i.e. engaging in metacognitive strategies, such as monitoring and reflecting on one’s own thought processes, can help individuals become more aware of cognitive processes and facilitate the identification and correction of misattributed memories.
Meditation is a form of metacognition because it involves the intentional observation, awareness, and regulation of one’s own cognitive and emotional processes. Through regular meditation practice, individuals can develop greater metacognitive skills, leading to improved self-awareness, emotional regulation, and cognitive control.Metacognition refers to the ability to monitor and regulate one’s own cognitive processes, and meditation practices often cultivate this ability in several ways: Observation of Thoughts: In meditation, practitioners are encouraged to observe their thoughts as they arise without judgment. This process of observing thoughts allows individuals to develop greater awareness of their cognitive processes, including patterns of thinking, biases, and distractions. Awareness of Emotions: Meditation practices often involve paying attention to emotions as they arise in the present moment. By acknowledging and accepting emotions without attachment or aversion, individuals can cultivate emotional awareness and regulate their responses more effectively. Focus and Attention: Many meditation techniques involve focusing attention on a specific object, such as the breath or a mantra. Through sustained attention practice, individuals can enhance their ability to regulate attention and become more mindful of distractions or wandering thoughts. Reflection and Insight: Meditation practices often facilitate self-reflection and insight into one’s own mental processes. Regular meditation can lead to a deeper understanding of oneself, including habitual patterns of thought and behavior, which can support metacognitive awareness and self-regulation. Cultivation of Presence: Meditation encourages individuals to cultivate a sense of presence and mindfulness in daily life. By being fully present in the moment, individuals can develop greater clarity of mind and awareness of their internal states, facilitating metacognitive processes.
Source confusion and misattribution of memory are related concepts in cognitive psychology, but they refer to slightly different phenomena. Misattribution of Memory: Misattribution occurs when an individual correctly remembers information but attributes it to the wrong source. For example, someone might hear a rumor from a friend but later remember it as something they read in a news article. The memory is accurate, but the source is misattributed. Misattribution can lead to false beliefs or inaccurate recollections about where information originated. Source Confusion: Source confusion, on the other hand, involves confusion about the origin of a memory itself. It occurs when a person is unable to distinguish whether a memory is something they experienced directly, something they imagined, or something they heard about from someone else. Source confusion can lead to the incorporation of false memories into one’s recollection of past events. For example, a person might have a vivid memory of a childhood event, but upon further examination, realize that the memory was actually a story they heard from a family member and not a personal experience.
Cryptomnesia is a psychological phenomenon where a person recalls something they have experienced or heard before but mistakenly believes it to be an original idea or creation. In essence, it involves unintentionally plagiarizing oneself. Previous Exposure: The individual is exposed to information or an experience in the past, whether consciously or unconsciously. This could be through reading, hearing, or observing something. Forgetting the Source: Over time, the individual forgets or fails to remember where they encountered the information or experience. The memory becomes stored in their mind without a clear recollection of its origin. Recreation as Original: Later on, the individual recalls or reproduces the information or experience, believing it to be a novel thought or creation. They may genuinely believe that they came up with the idea independently, unaware of its prior existence in their memory. Cryptomnesia can occur in various contexts, including creative endeavors such as writing, music composition, or invention. It can also occur in everyday conversations or discussions where individuals unintentionally repeat information they’ve previously heard without realizing they’re not the original source. In some cases, cryptomnesia can lead to accusations of plagiarism if others recognize the borrowed idea or content. However, cryptomnesia is generally considered to be unintentional and not an act of deliberate deception like plagiarism. To prevent cryptomnesia, it’s important for individuals to be mindful of their sources of information and to practice critical thinking and reflection to recognize when they may be drawing from previous experiences or knowledge. Additionally, proper citation and acknowledgment of sources can help mitigate the risk of unintentional plagiarism.
False memory refers to a phenomenon in which a person recalls events, experiences, or details that did not actually occur or were distorted from their original form. These memories can feel just as real and vivid as genuine memories, making it difficult for individuals to discern between what is true and what is false. Suggestion: External influences, such as leading questions, suggestive comments, or misinformation, can implant false memories in an individual’s mind. For example, through suggestive questioning, a person might come to believe they experienced an event that never actually happened. Misattribution: False memories can also arise from misattributing the source of information. For instance, a person might confuse a dream, imagination, or story they heard from someone else with an actual memory of an event they experienced. Confabulation: Confabulation occurs when the brain fills in gaps in memory with fabricated or distorted information without the individual’s awareness. This can happen due to brain damage, certain neurological conditions, or as a result of normal cognitive processes. Social Influence: Memories can be influenced by social factors, such as peer pressure or group conformity. In group settings, individuals may adopt or conform to the memories of others, leading to the formation of false memories. Emotional Influence: Emotions can also impact memory formation and retrieval. Strong emotions associated with an event may lead to the creation of false memories or the distortion of existing memories. False memories have been studied extensively in psychology and neuroscience. Research has shown that memory is not a perfect record of past events but is rather reconstructive in nature, subject to various biases and distortions. Understanding the mechanisms behind false memory formation is crucial for accurately assessing eyewitness testimony, understanding the reliability of autobiographical memories, and improving memory-related processes in various contexts.
By acknowledging and confronting implicit associations, individuals and organizations can work toward creating a more just and equitable world. Implicit association refers to the unconscious mental associations or biases that individuals hold about certain concepts, groups, or categories. These associations are formed automatically and unconsciously based on past experiences, cultural influences, and social conditioning. Implicit associations can influence attitudes, beliefs, perceptions, and behaviors, often without individuals being aware of them. The concept of implicit association is commonly studied in psychology using techniques such as the Implicit Association Test (IAT), which measures the strength of associations between different concepts by assessing response times to categorization tasks. For example, the IAT might measure the strength of associations between concepts like “good” and “bad” with categories like “white” and “black,” revealing any implicit biases individuals may hold.
Implicit associations can manifest in various domains, including race, gender, age, ethnicity, religion, sexual orientation, and social status. These associations can lead to implicit bias, where individuals unintentionally and unconsciously favor or disfavor certain groups or categories over others. Implicit bias can influence decision-making processes, interactions with others, and perceptions of individuals and groups. Recognizing and addressing implicit associations and biases is important for promoting fairness, equality, and inclusivity in society. Strategies for addressing implicit bias include increasing awareness of one’s own biases, challenging stereotypes and assumptions, fostering empathy and perspective-taking, promoting diversity and inclusion, and implementing bias-mitigation techniques in decision-making processes.
By acknowledging and addressing implicit stereotypes, individuals and organizations can work toward creating more equitable and inclusive environments. Implicit associations and implicit stereotypes are related concepts, but they refer to different aspects of social cognition: Implicit Associations: Implicit associations refer to automatic and unconscious mental connections or associations between concepts, categories, or attributes. These associations can be positive or negative and are formed based on past experiences, cultural influences, and social conditioning. Implicit associations can influence perceptions, attitudes, beliefs, and behaviors, often without individuals being aware of them. For example, an implicit association test (IAT) might measure the strength of associations between concepts like “good” and “bad” with categories like “white” and “black,” revealing any implicit biases individuals may hold.
Implicit Stereotypes: Stereotypes are cognitive schemas or mental representations of social groups, which are often based on oversimplified or exaggerated beliefs about the characteristics, traits, or behaviors of group members. Stereotypes can be explicit (consciously held and expressed) or implicit (unconscious and automatic). Stereotypes can influence perceptions, judgments, and behaviors toward members of specific social groups, leading to biased attitudes, discriminatory behaviors, and unequal treatment. For example, having an implicit stereotype about women being less competent as a driver or maths (or more competent as a cook) might influence recruitment decisions or candidate evaluations negatively (or positively) , even if the individuals consciously rejects the stereotype.
Implicit associations refer to unconscious mental connections between concepts, while stereotypes are cognitive representations of social groups that can influence attitudes and behaviors. Implicit associations can contribute to the formation and reinforcement of stereotypes, but they are not the same thing. Implicit associations are one factor that can contribute to the activation and application of stereotypes in social cognition. Unlike explicit stereotypes, which are consciously held beliefs about groups and are typically more overt and deliberate, implicit stereotypes operate at a subconscious level and may be activated automatically in various situations. Implicit stereotypes can affect how individuals perceive and interact with others, leading to biased judgments, decisions, and behaviors. Addressing implicit stereotypes requires increasing awareness of their existence and impact, challenging and confronting biased beliefs and assumptions, promoting diversity and inclusion, fostering empathy and perspective-taking, and implementing strategies to mitigate bias in decision-making processes.
Stereotypical bias refers to the tendency to make judgments or decisions about individuals based on stereotypes or generalized beliefs about their social group membership, rather than on individual characteristics, behaviors, or merits. It involves applying preconceived notions or assumptions about a group to individuals within that group, often leading to unfair or inaccurate evaluations. Generalization: Stereotypical bias involves making broad generalizations or assumptions about individuals based on their membership in a particular social group, such as race, gender, ethnicity, religion, sexual orientation, or socioeconomic status. Unconscious Influence: Stereotypical bias often operates at a subconscious level, meaning that individuals may not be consciously aware of the stereotypes influencing their judgments or decisions. These biases can occur automatically and unintentionally, even among individuals who reject stereotypes on a conscious level. Impact on Behavior: Stereotypical bias can influence various aspects of behavior, including perceptions, attitudes, beliefs, decisions, and actions. For example, it can affect hiring decisions, performance evaluations, academic assessments, criminal justice outcomes, and interpersonal interactions. Perpetuation of Inequality: Stereotypical bias can contribute to the perpetuation of inequality and discrimination within society by reinforcing existing power dynamics and privileging dominant groups over marginalized groups. It can lead to unequal treatment and opportunities for individuals based on their social group membership. Resistance to Change: Stereotypical bias can be resistant to change, even in the face of evidence contradicting stereotypes. Individuals may cling to stereotypes as a way of simplifying complex social realities or maintaining existing beliefs about the world. Addressing stereotypical bias requires increasing awareness of its existence and impact, challenging stereotypes and assumptions, promoting empathy and understanding across diverse groups, fostering critical thinking skills, and implementing strategies to mitigate bias in decision-making processes. By recognizing and addressing stereotypical bias, individuals and organizations can work toward creating more equitable and inclusive environments for al
Prejudice refers to preconceived opinions, attitudes, or feelings—usually negative—toward individuals or groups based on their perceived membership in a particular social category, such as race, ethnicity, gender, religion, sexual orientation, age, disability, or socioeconomic status. Prejudice often involves making unfair or unfounded judgments about others without considering individual differences or merits. Negative Attitudes: Prejudice involves holding negative attitudes, beliefs, or stereotypes about members of certain social groups. These attitudes may be based on misconceptions, misinformation, or cultural biases. Social Categorization: Prejudice is rooted in the tendency to categorize people into “us” versus “them” groups based on perceived differences. These categories can be based on visible characteristics such as race or gender, as well as more subtle factors like religion or political affiliation. Emotional Bias: Prejudice often involves an emotional component, such as fear, hostility, or dislike, toward members of the targeted group. These emotions may be fueled by underlying anxieties, insecurities, or societal pressures. Discriminatory Behavior: Prejudice can lead to discriminatory behavior, where individuals or groups are treated unfairly or unequally based on their membership in a particular social category. Discrimination can occur in various contexts, including employment, education, housing, healthcare, and criminal justice. Social Power: Prejudice is often intertwined with systems of power and privilege, where dominant groups maintain their status and advantage at the expense of marginalized or disadvantaged groups. This dynamic can perpetuate inequalities and injustices within society.
Prejudice is a complex and pervasive social phenomenon that can have profound consequences for individuals, groups, and societies as a whole. It undermines social cohesion, fosters division and conflict, and perpetuates inequality and injustice. Addressing prejudice requires challenging stereotypes, promoting empathy and understanding, fostering intergroup contact and cooperation, and advocating for policies and practices that promote equality and inclusion. For instance, throughout history, Jewish individuals and communities have faced prejudice, discrimination, and persecution based on their religious and ethnic identity. Anti-Semitism, which is hostility or prejudice against Jews, has been pervasive in various societies and cultures for centuries. Historical Persecution: Throughout history, Jews have been subjected to discrimination, persecution, and violence in many parts of the world. Examples include the Spanish Inquisition, pogroms in Eastern Europe, and the Holocaust during World War II, where six million Jews were systematically murdered by the Nazis. Stereotypes and Myths: Jewish people have often been stereotyped and scapegoated for societal problems, such as economic downturns or political unrest. Stereotypes portraying Jews as greedy, deceitful, or controlling have fueled anti-Semitic attitudes and behaviors. Restrictions and Exclusion: In various periods and regions, Jews have faced legal and social restrictions that limited their rights, opportunities, and freedoms. These restrictions included laws barring Jews from certain professions, living in certain areas, or marrying non-Jews. Discrimination in Education and Employment: Jewish individuals have encountered barriers to education and employment due to anti-Semitic attitudes and practices. They have been denied admission to schools and universities, excluded from certain professions, and subjected to discriminatory hiring practices. Violence and Hate Crimes: Jewish communities have been targeted by violence, hate crimes, and acts of terrorism motivated by anti-Semitic beliefs. Attacks on synagogues, Jewish schools, and other Jewish institutions have occurred in various countries around the world. Conspiracy Theories: Jewish people have been the subject of numerous conspiracy theories, such as the belief in a Jewish-controlled media or financial system. These conspiracy theories promote anti-Semitic attitudes and contribute to the spread of hate and misinformation. Despite these challenges, Jewish individuals and communities have made significant contributions to diverse fields including science, medicine, literature, arts, and philanthropy. Addressing anti-Semitism requires challenging stereotypes, promoting interfaith dialogue and understanding, and advocating for policies and practices that promote religious tolerance, equality, and respect for all individuals.
Another example of prejudice is the historical discrimination faced by African Americans in the United States during the era of segregation and Jim Crow laws. During this time, African Americans were systematically marginalized and oppressed by laws, policies, and societal norms that enforced racial segregation and upheld white supremacy. As a result, African Americans experienced widespread discrimination and inequality in various aspects of life, including: Segregated Facilities: Public facilities such as schools, transportation, restaurants, theaters, and even drinking fountains were segregated, with separate facilities for white and black individuals. African Americans were often denied access to or forced to use inferior facilities. Limited Opportunities: African Americans faced significant barriers to education, employment, housing, and political participation. They were often denied access to quality schools, job opportunities, and housing in predominantly white neighborhoods. Violence and Intimidation: African Americans were subjected to violence, intimidation, and threats from white supremacist groups such as the Ku Klux Klan. Lynchings, bombings, and other acts of racial violence were used to maintain white supremacy and intimidate African American communities. Voting Rights Suppression: African Americans were systematically disenfranchised through tactics such as poll taxes, literacy tests, and grandfather clauses, which were designed to prevent them from exercising their right to vote. Negative Stereotypes: African Americans were subjected to negative stereotypes and caricatures in the media, literature, and popular culture, which perpetuated racist beliefs and attitudes. This example illustrates how prejudice and systemic racism can intersect to create profound and enduring inequalities within society. The legacy of this historical prejudice continues to impact African American communities today, contributing to disparities in areas such as education, employment, healthcare, and criminal justice. Addressing prejudice requires acknowledging and confronting the historical injustices faced by marginalized groups and working towards systemic change to promote equality and social justice for all individuals.
The curse of knowledge bias can be associated with several principles, primarily those related to improving communication, simplifying systems, and considering the needs and perspectives of users. While this primarily focused on technical problem-solving, its principles can also be applied to address cognitive biases and improve human-centered aspects of technical systems. This principle involves designing systems that allow users to perform tasks or access information independently. To mitigate the curse of knowledge bias, self-service can be applied to provide users with resources, tools, and support mechanisms that empower them to learn and troubleshoot technical issues on their own, reducing their reliance on experts and minimizing the impact of communication barriers.
The generation effect is a phenomenon in cognitive psychology where individuals demonstrate better memory and retention of information that they actively generate or produce themselves compared to information that they passively receive. In other words, when individuals engage in the process of generating information, such as by answering questions, solving problems, or creating examples, they are more likely to remember that information later on. Key aspects of the generation effect include: Active Engagement: The generation effect occurs when individuals actively engage with the material, such as by retrieving it from memory, rather than passively receiving it. Encoding Specificity: When individuals generate information themselves, it becomes more tightly integrated into their existing knowledge networks, making it easier to retrieve later on. Enhanced Memory: Studies have shown that actively generating information leads to better memory retention and recall compared to simply reading or listening to information. Application to Learning: The generation effect has implications for educational practices, suggesting that activities that require students to actively generate information, such as self-testing, problem-solving, and teaching others, can be more effective for promoting learning and retention. Overall, the generation effect highlights the importance of active engagement and participation in the learning process for optimizing memory and retention. By actively generating information, individuals not only deepen their understanding of the material but also enhance their ability to remember it over time.
Belief bias is a cognitive bias where individuals’ evaluation of the validity of an argument is influenced by the believability of its conclusion rather than by the strength of the argument itself. In other words, people tend to accept arguments that align with their existing beliefs or expectations, even if those arguments are logically flawed or weak, while rejecting arguments that contradict their beliefs, even if those arguments are logically sound. Key characteristics of belief bias include: Influence of Prior Beliefs: Belief bias occurs when individuals’ prior beliefs or attitudes influence their reasoning process. People are more likely to accept arguments that support their existing beliefs and reject arguments that challenge them. Confirmation Bias: Belief bias is closely related to confirmation bias, which is the tendency to seek out, interpret, and remember information in a way that confirms one’s preexisting beliefs. Belief bias reflects the impact of confirmation bias on the evaluation of logical arguments. Weakness in Critical Thinking: Belief bias can lead to errors in judgment and decision-making by causing individuals to prioritize belief consistency over logical consistency. This can result in the acceptance of invalid arguments and the rejection of valid arguments. Resistance to Counterarguments: Individuals may be resistant to considering or engaging with arguments that contradict their beliefs, leading to a reluctance to critically evaluate opposing viewpoints and a tendency to dismiss them outright. Belief bias has important implications for reasoning, argumentation, and persuasion. It highlights the role of cognitive biases in shaping individuals’ evaluation of information and their susceptibility to persuasion. By being aware of belief bias, individuals can strive to approach arguments and evidence with greater objectivity and open-mindedness, considering the strength of the argument itself rather than the believability of its conclusion. This can help mitigate the influence of belief bias on decision-making and promote more rational and evidence-based reasoning.
Egocentric bias is a cognitive bias where individuals tend to rely too heavily on their own perspective when making judgments or interpreting information, while failing to adequately consider the perspectives of others. This bias can lead people to overestimate the extent to which others share their beliefs, attitudes, or knowledge, and to underestimate the influence of situational factors on other people’s behavior. Here are some key features of egocentric bias: Limited Perspective Taking: People affected by egocentric bias may struggle to take the perspective of others or to accurately imagine how others might perceive a situation. As a result, they may assume that others think and feel similarly to themselves, even when this is not the case. Projection of Own Experiences: Individuals may project their own thoughts, feelings, and motivations onto others, assuming that others are motivated by the same factors that drive their own behavior. This can lead to misunderstandings and misinterpretations of other people’s actions. Attribution Errors: Egocentric bias can also manifest in attribution errors, where individuals attribute their own successes to internal factors, such as skill or effort, while attributing their failures to external factors, such as luck or situational constraints. This bias can lead people to make inaccurate judgments about the behavior and intentions of others.
Impact on Communication and Relationships: Egocentric bias can have negative consequences for communication and relationships, as it can lead to misunderstandings, conflicts, and a lack of empathy or understanding for others’ perspectives. People affected by egocentric bias may struggle to see situations from the viewpoints of others, making it difficult to communicate effectively or resolve conflicts. To mitigate egocentric bias, individuals can: Practice perspective-taking exercises to better understand and empathize with the viewpoints of others. Seek out diverse perspectives and actively listen to the experiences and opinions of others. Challenge assumptions and biases by considering alternative explanations for behavior and outcomes. Cultivate self-awareness and mindfulness to recognize when egocentric biases may be influencing judgments and decisions. By being mindful of egocentric bias and taking steps to mitigate its effects, individuals can improve their interpersonal relationships, communication skills, and ability to navigate social interactions effectively.
The Dunning-Kruger effect is a cognitive bias where individuals with low ability or knowledge in a particular domain tend to overestimate their competence and skills, while those with higher ability or expertise tend to underestimate their competence. In other words, people who are less competent in a task are often unaware of their incompetence and may mistakenly believe they are more skilled or knowledgeable than they actually are. Key aspects of the Dunning-Kruger effect include: Incompetent Individuals Overestimating Abilities: People with low competence in a given area may lack the skills or knowledge to accurately assess their own performance. As a result, they may overestimate their abilities and believe they are performing better than they actually are. This overestimation can be fueled by a lack of metacognitive awareness or the inability to recognize one’s own mistakes or limitations.
Competent Individuals Underestimating Abilities: Conversely, individuals with higher levels of competence may underestimate their abilities due to their awareness of the complexity and nuances of the task. They may be more likely to recognize gaps in their knowledge or skills and to have a more accurate understanding of their limitations. This tendency to underestimate competence may also be influenced by humility or self-reflection. Impact on Learning and Decision Making: The Dunning-Kruger effect can have significant implications for learning, decision-making, and performance. For example, individuals who overestimate their abilities may be less motivated to seek out feedback or improve their skills, leading to stagnation or suboptimal performance. Conversely, those who underestimate their abilities may be overly cautious or hesitant to take on new challenges, limiting their potential for growth and development.
Mitigating the Effect: To mitigate the impact of the Dunning-Kruger effect, individuals can: Seek out objective feedback and evaluation from trusted sources to gain a more accurate understanding of their performance. Engage in ongoing learning and skill development to improve competence and expertise in areas of interest. Cultivate self-awareness and humility to recognize and acknowledge gaps in knowledge or skills. Encourage a culture of constructive feedback and reflection in educational and professional settings to support continuous improvement. By being aware of the Dunning-Kruger effect and taking steps to mitigate its influence, individuals can make more informed decisions, improve their performance, and foster a growth mindset conducive to learning and development.
The self-relevance effect is a cognitive bias where individuals demonstrate superior memory for information that is personally relevant to themselves compared to information that is less personally relevant or unrelated. This bias reflects the tendency for people to prioritize and better remember information that has direct relevance to their own experiences, beliefs, values, or identity. Key aspects of the self-relevance effect include: Enhanced Encoding and Processing: Information that is perceived as personally relevant is more likely to be encoded deeply and processed elaborately compared to irrelevant information. This enhanced encoding and processing result in stronger memory traces and better retention of self-relevant information over time. Emotional and Motivational Significance: Self-relevant information often carries emotional and motivational significance for individuals, leading to heightened attention, arousal, and engagement during encoding. This emotional and motivational significance can enhance memory consolidation and retrieval processes, making self-relevant information more salient and memorable. Autobiographical Memory: The self-relevance effect is closely related to autobiographical memory, which involves the recall of personally experienced events and episodes from one’s own life. Self-relevant information is often integrated into individuals’ autobiographical memory and contributes to their sense of identity and self-concept. Real-World Implications: The self-relevance effect has practical implications for various aspects of everyday life, including education, marketing, and interpersonal communication. Educators can leverage self-relevant learning materials and examples to enhance student engagement and memory retention. Similarly, marketers can tailor advertising messages to resonate with consumers’ self-concepts and values to increase message effectiveness and memorability. By understanding the self-relevance effect and its influence on memory processes, individuals and organizations can leverage the power of self-relevant information to enhance learning, communication, and decision-making. Recognizing the importance of self-relevance can help optimize the design of educational materials, marketing campaigns, and communication strategies to better resonate with target audiences and improve memory performance.
Just World Hypothesis is the belief that people get what they deserve and deserve what they get. In a technical context, this bias might lead designers or problem solvers to attribute technical failures or challenges to personal shortcomings rather than systemic issues or external factors. This could result in a lack of accountability for design flaws or an underestimation of the complexity of technical problems.
Not Invented Here bias refers to the tendency of individuals to reject external ideas or solutions in favor of those developed internally. In designing a technical system, the not invented here bias might lead to a reluctance to adopt proven technologies or best practices developed by external sources, limiting innovation and potentially resulting in the reinvention of the wheel. When solving technical problems, this bias might prevent individuals from considering alternative solutions proposed by external experts or organizations.
Reactive Devaluation occurs when individuals devalue proposals or solutions simply because they originated from a perceived adversary or competitor. In a technical context, this bias might lead designers or problem solvers to dismiss potentially valuable ideas or solutions simply because they come from rival companies or individuals. This could result in missed opportunities for collaboration or the adoption of superior technologies.
Social Desirability Bias: Social desirability bias occurs when individuals provide responses that they believe are socially acceptable or desirable rather than expressing their true opinions or beliefs. In a technical context, this bias might lead designers or engineers to prioritize features or solutions that align with prevailing norms or expectations, potentially overlooking alternative approaches or user needs. When solving technical problems, individuals might be reluctant to acknowledge uncertainties or limitations, leading to overconfident or unrealistic assessments of the situation.
Overconfidence Effect: The overconfidence effect refers to the tendency for individuals to overestimate their own abilities, knowledge, or predictions. In a technical context, this bias might lead designers or engineers to be overly confident in the performance or reliability of their designs or solutions, potentially overlooking potential flaws or vulnerabilities. When solving technical problems, individuals might underestimate the complexity or difficulty of the problem, leading to overambitious or unrealistic expectations for problem resolution.
Ultimate Attribution Error: The ultimate attribution error is the tendency to attribute positive actions or outcomes of one’s in-group to internal factors (such as character or ability) and negative actions or outcomes of out-groups to external factors (such as circumstances or luck). In a technical context, this bias might lead designers or engineers to attribute the success of their own team or organization to internal factors such as skill or competence, while attributing the failure of rival teams or organizations to external factors such as incompetence or luck. This bias can result in a lack of recognition for the contributions of external parties or an underestimation of their capabilities.
Cross-Race Effect: The cross-race effect, also known as the own-race bias or the other-race effect, is the tendency for individuals to better recognize and remember faces of individuals from their own racial or ethnic group compared to faces of individuals from other racial or ethnic groups. In designing a technical system, this bias might lead to disparities in facial recognition algorithms or image processing systems, where individuals from certain racial or ethnic groups are more accurately identified or represented than others. When solving technical problems, this bias might result in the underrepresentation or misclassification of individuals from minority groups, leading to biased outcomes or decisions.
2: Mass of the non-moving object: [’37: Complexity of control and measurement’]
4: Length of the non-moving object: [’18: Brightness, Visibility’, ’33: Convenience of use’]
7: Volume of the moving object: [’28: Accuracy of measurement’, ’29: Accuracy of manufacturing’]
8: Volume of the non-moving object: [’29: Accuracy of manufacturing’]
9: Speed: [’29: Accuracy of manufacturing’]
10: Force: [’33: Convenience of use’]
11: Tension, Pressure: [’22: Energy loss’, ’28: Accuracy of measurement’]
12: Shape: [’15: Action time of the moving object’]
14: Strength: [’33: Convenience of use’, ’36: Complexity of the structure’]
15: Action time of the moving object: [’12: Shape’]
16: Action time of the non-moving object: [’37: Complexity of control and measurement’]
17:Temperature: [’21: Power’]
18: Brightness, Visibility: [’39: Productivity’]
19: Energy consumption of the moving object: [‘5: Area of the moving object’, ’11: Tension, Pressure’]
20: Energy consumption of the non-moving object: [’37: Complexity of control and measurement’]
21: Power: [‘8: Volume of the non-moving object’, ’17:Temperature’]
22: Energy loss: [’26: Amount of substance’]
26: Amount of substance: [’22: Energy loss’, ’33: Convenience of use’, ’34: Convenience of repair’]
27: Reliability: [’15: Action time of the moving object’]
28: Accuracy of measurement: [‘2: Mass of the non-moving object’, ’32: Convenience of manufacturing’]
29: Accuracy of manufacturing: [‘8: Volume of the non-moving object’, ’34: Convenience of repair’]
30: Harmful external factors: [’33: Convenience of use’]
33: Convenience of use: [‘1: Mass of the moving object’, ‘2: Mass of the non-moving object’, ’15: Action time of the moving object’, ’16: Action time of the non-moving object’, ’28: Accuracy of measurement’, ’30: Harmful external factors’]
34: Convenience of repair: [‘3: Length of the moving object’, ‘6: Area of the non-moving object’, ‘7: Volume of the moving object’, ’25: Time loss’, ’26: Amount of substance’, ’29: Accuracy of manufacturing’]
37: Complexity of control and measurement: [’15: Action time of the moving object’, ’16: Action time of the non-moving object’]
38: Level of automation: [’14: Strength’, ’37: Complexity of control and measurement’]
39: Productivity: [’34: Convenience of repair’]
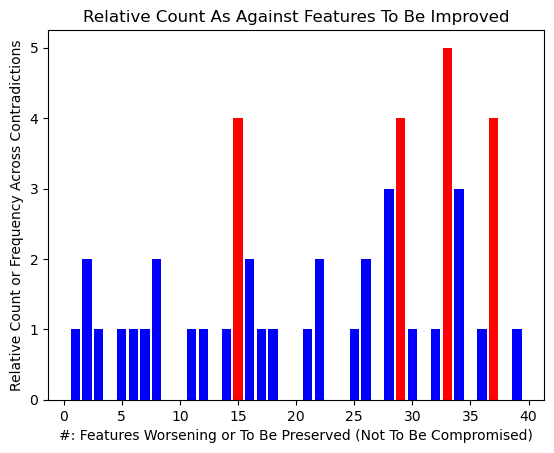
2/37 4/18 4/33 7/28 7/29 8/29 9/29 10/33 11/22 11/28 12/15 14/33 14/36 15/12 16/37 17/21 18/39 19/5 19/11 20/37 21/8 21/17 22/26 26/22 26/33 26/34 27/15 28/2 28/32 29/8 29/34 30/33 33/1 33/2 33/15 33/16 33/28 33/30 34/3 34/6 34/7 34/25 34/26 34/29 37/15 37/16 38/14 38/37 39/34
EXAMPLE: Traditional banking systems relied heavily on bank staff to handle routine transactions. Traditional bank branches had fixed operating hours, limiting customer access. Bank branches often experience long queues, leading to delays in customer service. Customers faced challenges accessing their banks, especially in remote or distant locations. By introducing self-service concept, Automated Teller Machines (ATMs) addresses several challenges associated with traditional banking, providing customers with increased accessibility, convenience, and flexibility in managing their finances. ATMs have now become an integral part of modern banking systems, enhancing the overall efficiency and customer experience. By providing basic banking services, ATMs help reduce traffic at physical bank branches. This means that bank staff can focus on more complex customer needs, leading to improved productivity in the branches.
Contradiction (39/34): Increase the productivity and reach of banking operations using information technologies (39) while also making it easy to operate or diagnose or repair the system (34).
Solution: An ATM is a prime example of a self-service system. ATMs offer a quick and efficient alternative, reducing wait times. ATMs provide ubiquitous access (24/7 accessibility), overcoming geographical constraints.It allows users to perform various banking transactions without the need for direct interaction with bank staff. ATMs provide round-the-clock access to banking services, allowing customers to perform transactions at any time. ATMs are designed to process transactions quickly, allowing users to withdraw cash, check balances, transfer funds or perform other banking activities efficiently. This speed enhances the overall productivity of the banking process, reducing waiting times for customers. This extended availability enhances customer convenience and increases the overall productivity of banking services. ATMs reduce this dependency, allowing staff to focus on more complex customer needs. ATMs provide users with a level of privacy when conducting transactions, which might be preferable for tasks like checking balances or withdrawing cash. ATMs offer a convenient and time-efficient means of conducting basic banking transactions without the need for face-to-face interactions. In emergencies or urgent situations.
ATMs provide immediate access to cash and essential banking services when traditional branches might be closed. ATMs contribute to cost savings for banks by automating routine transactions, reducing the need for additional staff and operational costs. Users can withdraw cash from their bank accounts using the ATM without visiting a physical bank branch. Customers can check their account balances at any time, providing real-time information about their financial status. Some ATMs allow users to deposit cash or checks, offering a convenient alternative to visiting a bank teller. ATMs enable users to transfer funds between their accounts, facilitating basic financial transactions. Users can obtain mini statements, providing a summary of recent transactions without visiting the bank. Some ATMs support bill payments, allowing users to settle utility bills and other payments electronically.
By combining enhanced productivity for users with advanced maintenance features, ATMs exemplify a technology that not only benefits customers but also offers operational efficiency and ease of maintenance for financial institutions. The integration of technology and proactive maintenance strategies contributes to a more robust and reliable ATM network. Modern ATMs often come with remote monitoring capabilities. This allows banks to monitor the status of ATMs, perform diagnostics, and identify issues without sending technicians on-site. Remote management enhances maintenance efficiency. ATMs are equipped with self-diagnostic tools that can identify common issues and errors. This functionality helps in quick issue resolution and minimizes the need for extensive manual troubleshooting. Many ATMs have a modular design, meaning that individual components can be easily replaced or upgraded without affecting the entire machine. This modularity simplifies maintenance tasks and reduces downtime. Some ATMs use predictive maintenance algorithms that analyze usage patterns and component health to predict potential failures. This proactive approach enables scheduled maintenance, minimizing unplanned downtime. Software updates and patches can be deployed remotely to ATMs. This allows banks to implement security enhancements, new features, or bug fixes without the need for physical intervention, reducing maintenance costs. Banks often use centralized management systems to monitor and control a network of ATMs. This centralization streamlines maintenance processes, as updates, configurations, and troubleshooting can be managed from a central location. Collaborations with ATM vendors often include maintenance and support agreements. These partnerships ensure that expert technicians are available for timely repairs, reducing the impact of technical issues on ATM availability.