15: DYNAMICITY (Dynamization, Relative Motion): (A) Alter or adjust the characteristics of an object (or system or process) or its external environment, to gain optimal performance at each stage of its operation, (B) make an immobile or rigid object (or system or process), movable or interchangeable (or adjustable/adaptable/flexible), (C) Divide an object into elements capable of changing their position relative to each other
SYNONYMS: Dynamization, Relative Motion, Configurability, Customization/Personalization, Multiplicity, Transition To Micro-Level. Miniaturization
EXAMPLE: Adjustable Mirrors, Steering Wheel and Seats in Vehicles, Multi-Step Transformer, Toothbrush Bristles, Drinking Straws, Road Dividers, “Butterfly” Computer Keyboard, Scissors, Foldable Knife, Retractable Aircraft landing Gear, Smart Thermostats, Personlized Software Applications, Dynamic Routing, Dynamic Pricing, Boroscope, Sigmoidoscope, Food Trucks,
ACB:
The “Dynamicity” principle is applied to create systems, materials, or processes that can adapt, change, or optimize themselves based on external factors. This adaptability enhances performance, efficiency, and the ability to address contradictions in complex systems. It refers to the ability of a system or solution to change or adapt dynamically in response to different conditions or requirements. It involves designing systems that can alter their behavior, structure, or properties based on external stimuli or changing circumstances.
It could fundamentally also means transitioning to micro-level by increasing the depth or span of controllability and hence improves the configurabiity or adaptibility or flexibility of the system. Have more paramters of concern open as options as that you can use to configure a product or service or system and introduce more dynamicity or mulltiple of outcome or effect. Hence it allows the system to adapt to the optimal or different requirements or scenarios. Transitioning to micro level is part of the laws of evolution of system and is somewhat related to the prinicple of dynamicity as well as by introducing more levels of control or increasing the depth or span of control by going to the minimum or smallest level in terms of part or component of the system, it helps change the behaviour of the system. One can introduce variability in the behaviour of a system by zooming in (from internal most part or component to outermost or external or super system) or zooming out (from external or super system to internal most or smallest configurable sub-system in the hiearchical or horizontal breakdown architecture of a system).
Fan and light regulators embody the dynamism principle by introducing variability, adaptability, and responsiveness into the operation of these systems. By enabling users to adjust the speed or intensity according to their preferences and needs, regulators enhance comfort, efficiency, and control while addressing potential contradictions such as energy consumption and environmental impact. However, challenges such as compatibility issues, reliability concerns, or complexity in user interfaces may arise, requiring careful design and implementation to ensure optimal performance and user satisfaction.
Fan or light regulators, which control the speed of a fan or the intensity of light, exemplify the dynamism principle in several ways: Variability in Operation: Fan or light regulators allow users to adjust the speed of a fan or the intensity of light according to their preferences or needs. By providing a range of settings, these regulators introduce variability into the system’s operation, enabling it to respond dynamically to changing environmental conditions or user requirements. Feedback Mechanisms: Many modern fan or light regulators incorporate feedback mechanisms that continuously monitor and adjust the system’s performance based on input from sensors or user commands. These feedback loops enable real-time adjustments to optimize efficiency, comfort, or energy consumption, enhancing the system’s dynamism and responsiveness.
Adaptation to Changing Conditions: Fan or light regulators enable users to adapt the system’s operation to changing conditions, such as temperature variations or daylight levels. For example, a fan regulator allows users to increase the fan speed to cool a room more quickly on hot days or decrease it to maintain a comfortable temperature during cooler periods. Similarly, a light dimmer enables users to adjust the brightness of a light fixture to suit different tasks or ambient lighting conditions. Energy Efficiency: By allowing users to adjust the speed of a fan or the intensity of light, regulators can help optimize energy consumption and reduce operating costs. For instance, lowering the fan speed or dimming the lights when full power is not needed can save energy and prolong the lifespan of the equipment. This aligns with the principle of dynamism by promoting efficient resource utilization and adaptation to changing energy requirements.
The “Principle of Transition to a Micro-Level” and the “Dynamicity” principle are distinct concepts. Principle of Transition to a Micro-Level involves moving from a macro-level to a micro-level, often emphasizing miniaturization and working with components or processes at a smaller scale to achieve specific benefits in general. The Dynamicity principle refers to making a system or object more dynamic or capable of changing its properties, states, or configurations. It involves introducing movement, variability, or adaptability into a system. While both principles may involve changes or transitions, they focus on different aspects as such. Transition to Micro Level is more about scaling down to a smaller level, while Dynamicity is more about introducing dynamism, adaptability, or variability into a system (and that can also be achieved by transitioning to micro level as on of the ways). They can be applied independently or in conjunction, depending on the specific problem or contradiction being addressed.
The “Dynamicity” principle is applied to create systems, materials, or processes that can adapt, change, or optimize themselves based on external factors. This adaptability enhances performance, efficiency, and the ability to address contradictions in complex systems. It refers to the ability of a system or solution to change or adapt dynamically in response to different conditions or requirements. It involves designing systems that can alter their behavior, structure, or properties based on external stimuli or changing circumstances.
It could fundamentally also means transitioning to micro-level by increasing the depth or span of controllability and hence improves the configurabiity or adaptibility or flexibility of the system. Have more paramters of concern open as options as that you can use to configure a product or service or system and introduce more dynamicity or mulltiple of outcome or effect. Hence it allows the system to adapt to the optimal or different requirements or scenarios. Transitioning to micro level is part of the laws of evolution of system and is somewhat related to the prinicple of dynamicity as well as by introducing more levels of control or increasing the depth or span of control by going to the minimum or smallest level in terms of part or component of the system, it helps change the behaviour of the system. One can introduce variability in the behaviour of a system by zooming in (from internal most part or component to outermost or external or super system) or zooming out (from external or super system to internal most or smallest configurable sub-system in the hiearchical or horizontal breakdown architecture of a system).
The Pattern of Dynamization, is also one of the laws of evolution of a technical system and it directly refers this principle of dynamicity i.e. moving from a rigid system to a flexible structure or system. This transition involves introducing joints or movable elements into a system, leading to increased flexibility. The process can be summarized by adding flexibility gradually, making the entire system more adaptable. A rigid steering column in a car to introduction of a joint allowing vertical adjustment leading to a flexible steering column that can be adjusted. From a fixed antenna to making the antenna collapsible to a flexible antenna that can be folded. from a fixed landing gear to designing landing gear that can fold and retract to flexible landing gear for improved aerodynamics. In these examples, the evolution involves introducing joints or flexible elements, allowing the system to adapt, adjust, or retract as needed. This enhances the overall flexibility and functionality of the technical systems.
Adaptive cruise control systems in modern vehicles dynamically adjust the speed to maintain a safe following distance from the vehicle ahead. The system constantly adapts to changes in traffic conditions, slowing down or accelerating as needed. Smart thermostats dynamically adjust heating or cooling settings based on user preferences, daily routines, and real-time environmental conditions. They learn and adapt over time to optimize energy efficiency. Lighting systems that automatically adjust brightness and color temperature based on natural light conditions and user preferences. This dynamic adaptation enhances visual comfort and energy efficiency. Materials that can change their shape or properties in response to external stimuli, such as temperature or pressure changes. For example, shape memory alloys dynamically shift back to their original form when heated. Websites with dynamic content that adjusts based on user interactions, preferences, or real-time data. Dynamic websites provide personalized experiences and adapt to user behavior. Dynamic routing protocols in computer networks adjust the paths data takes based on real-time network conditions. This ensures efficient data transmission and adapts to changes in the network topology.
Dynamic stability control systems in vehicles adjust brake and engine forces to maintain stability during sudden maneuvers or slippery conditions. The system dynamically responds to changes in the vehicle’s behavior. Educational platforms that dynamically adapt learning content based on individual progress, preferences, and performance. These systems provide personalized learning experiences. E-commerce platforms that dynamically adjust product prices based on factors like demand, competition, or user behavior. Dynamic pricing systems respond in real-time to market conditions. Agile methodologies in project management involve dynamic adaptation to changing project requirements. Teams continuously reassess priorities and adjust their approach based on feedback and evolving circumstances.
By making the traffic management system dynamic, adaptive, and configurable, cities can effectively manage urban traffic flow, improve safety, and enhance the overall efficiency of transportation networks. A modern traffic management system dynamically adapts to changing traffic conditions in real-time. It uses various sensors, cameras, and data analytics to monitor traffic flow, congestion levels, and incidents such as accidents or road closures. By collecting and analyzing this data, the system can dynamically adjust traffic signal timings, lane configurations, and routing strategies to optimize traffic flow and minimize congestion. Traffic management systems offer configurability to accommodate different traffic scenarios and user preferences. For example, city planners can configure the system to prioritize certain types of vehicles (e.g., emergency vehicles, public transit) or to implement specific traffic management strategies during peak hours or special events. Users may also have the option to configure personalized routing preferences or receive real-time traffic updates through mobile applications or navigation systems. The operation of a traffic management system changes dynamically over time to reflect daily traffic patterns, seasonal variations, and special events. For instance, the system may adjust signal timings during rush hours to prioritize traffic flow along major commuting routes. It may also implement temporary changes, such as road closures or detours, to accommodate construction projects or large-scale events like parades or marathons.
Dynamic adaptation allows the system to respond quickly to changing traffic conditions, reducing congestion and travel times for commuters. Configurable features such as adaptive signal timings and lane configurations can improve intersection safety and reduce the risk of accidents. By dynamically allocating resources such as traffic signals and lane assignments based on real-time data, the system optimizes the use of infrastructure and minimizes energy consumption.
At an abstract level, the “Dynamicity” principle refers to the capability of a system, process, or solution to change, adapt, or respond dynamically to varying conditions, stimuli, or requirements. It emphasizes the importance of designing systems that can exhibit flexibility, responsiveness, and the ability to evolve over time. Designing systems that can adapt their behavior, structure, or properties based on changing external factors or inputs. Implementing mechanisms that allow a system to adjust its parameters in real-time, ensuring optimal performance under different conditions. Creating systems that can continuously learn, evolve, and improve their performance over time. Incorporating feedback loops, learning algorithms, or adaptive processes that enable the system to refine its functionality based on past experiences and new information.
Making a chair revolving addresses the contradiction between the need for a stationary sitting position and the desire for flexibility and easy movement.Traditional chairs are stationary and limit the flexibility of movement for the person sitting in them. This may hinder easy access to different areas around the desk or workspace. There is a trade-off between maintaining a fixed seating position for stability and allowing the user the flexibility to easily reach various parts of the surrounding space. The introduction of a revolving feature to the chair aligns with the principle of “Dynamicity” and “Curvature”. This principle involves transitioning from a rigid to a flexible structure, allowing for increased adaptability and ease of movement. By incorporating a swivel or revolving mechanism into the chair’s design, individuals can easily rotate or turn the chair to reach different areas without having to stand up. This solution provides a compromise between the need for stability while sitting and the desire for flexibility in movement. In short, making a chair revolving resolves the contradiction between stationary seating and the need for flexible movement, enhancing the user’s convenience and accessibility in various working environments.
Providing systems with the ability to exhibit versatility and flexibility in their operations. Designing solutions that can handle a range of inputs, tasks, or requirements without significant reconfiguration, enabling them to address diverse scenarios. Enabling systems to make adjustments in real-time, responding instantly to changes in the environment or user needs. Integrating sensors, feedback mechanisms, or intelligent algorithms that allow the system to dynamically respond to evolving conditions without delays.
Designing systems with a degree of autonomy and self-organization to manage and optimize their own processes. Implementing self-regulating mechanisms, self-healing algorithms, or autonomous decision-making components that enable the system to organize and optimize its functions independently. Developing systems that can navigate and thrive in uncertain or unpredictable environments. Creating solutions with built-in resilience, redundancy, or adaptability to handle unforeseen challenges and uncertainties. Maximizing the efficient use of resources by allowing systems to dynamically allocate, redistribute, or optimize resources based on changing demands. Implementing resource management strategies that adapt to variations in workload, prioritizing and allocating resources dynamically.
Ensuring that systems have the capacity to evolve and be upgraded to incorporate new features, technologies, or capabilities. Designing modular architectures or upgradable components that facilitate the seamless integration of advancements without major overhauls. Focusing on systems that can adapt to user preferences, behaviors, or needs. Building interfaces, applications, or products that dynamically adjust their features, content, or interactions based on user feedback and usage patterns.
Dynamicity principles address technical and business contradictions by enabling systems and processes to adapt, learn, and optimize based on changing technical or business requirements, ultimately fostering resilience, efficiency, and innovation. For example: (a) Ensuring optimal energy usage while maintaining a comfortable indoor environment. Implementing smart building systems that dynamically adjust heating, cooling, and lighting based on occupancy, external weather conditions, and energy demand. (b) Balancing the need for efficiency with the unpredictability in the supply chain. Utilizing dynamic routing algorithms and real-time tracking to adapt supply chain routes based on traffic, weather, and unforeseen disruptions.
Continuous monitoring without intrusiveness. Developing wearable health devices that dynamically adapt measurement intervals based on user activity, optimizing battery life without compromising data accuracy. Balancing high-volume production with the need for customization. Implementing adaptive manufacturing systems that can reconfigure production lines based on real-time demand and customer preferences. Precision surgery without real-time adjustments. Integrating robotic surgery systems with real-time feedback mechanisms that adapt to patient movement, ensuring continuous precision during surgery.
Meeting project timelines while accommodating changing requirements. Adopting agile methodologies that allow for dynamic adjustments to project scope and priorities based on evolving customer needs and feedback. Enhancing customer engagement without intrusive tactics.Implementing dynamic pricing strategies, personalized promotions, and interactive displays that adapt to customer preferences and behaviors. Balancing competitiveness with consistent pricing. Implementing dynamic pricing algorithms that adjust product prices in real-time based on demand, competition, and market trends.
Creating effective marketing strategies for diverse customer segments. Utilizing data-driven marketing campaigns that dynamically adjust content, channels, and timing based on individual customer behaviors and preferences. Ensuring employee retention while adapting to changing workforce expectations. Implementing flexible work policies, continuous learning programs, and career development plans that adapt to individual employee needs and aspirations.
Providing transparency without overwhelming data. Implementing dynamic supply chain visibility platforms that selectively present relevant information based on user roles, reducing information overload. Balancing efficiency with personalized service. Integrating AI-driven chatbots and dynamic customer support systems that adapt responses based on customer inquiries, improving efficiency while maintaining a personalized touch.
Morphological Analysis is a problem-solving and decision-making method that was developed by Fritz Zwicky, a Swiss scientist and engineer, in the 1940s. Fritz Zwicky was an astrophysicist and aerospace engineer who made significant contributions to various scientific fields, including the development of morphological analysis. Morphological Analysis involves creating a multidimensional matrix that represents the various dimensions or factors related to a complex problem. In the matrix, different parameters or factors contributing to the problem are listed along the rows, and the potential states or options for each parameter are listed along the columns. The method systematically explores the combinations of different states for each parameter, resulting in a comprehensive overview of possible solutions or scenarios. A cross-consistency check is performed to ensure that the combinations are logically feasible and do not violate any constraints. The method helps in identifying potential solutions by evaluating and selecting combinations that meet the desired criteria or objectives.
Morphological Analysis provides a structured and systematic approach to exploring complex problems by breaking them down into manageable dimensions. By considering various combinations of states for each parameter, the method helps in creating a comprehensive solution space, revealing options that might not be apparent in a more linear analysis. The method allows for the identification of trade-offs and dependencies between different factors, helping decision-makers understand the implications of different choices. Morphological Analysis facilitates objective decision-making by organizing information in a clear matrix, making it easier to evaluate and compare different solutions.
Originally developed in the context of engineering and design, Morphological Analysis has been applied in various multidisciplinary fields, including business, policy, and science. The method encourages creative thinking by exploring a wide range of possibilities and potential combinations. Morphological Analysis is particularly useful in situations where problems are multifaceted, involving numerous variables and dimensions. It provides a systematic way to navigate complex problem spaces and arrive at informed decisions or solutions. While it was initially applied in aerospace engineering, its adaptability has led to its use in various domains for structured problem-solving.
The concept of time-sharing in computing was developed independently by multiple researchers and institutions during the late 1950s and early 1960s. The time-sharing model refers to a computing model in which multiple users have simultaneous access to a single computer. This is achieved by rapidly switching the attention of the central processing unit (CPU) between different tasks or users. Each user gets a small portion of the CPU’s time, allowing them to interact with the computer. One of the early pioneers in time-sharing systems was John McCarthy, who worked on the Compatible Time-Sharing System (CTSS) at the Massachusetts Institute of Technology (MIT) in the early 1960s. Another significant contribution came from researchers at Dartmouth College who developed the Dartmouth Time-Sharing System (DTSS) in the late 1960s. Additionally, Christopher Strachey at the University of Oxford played a role in the development of time-sharing ideas. While no single individual can be credited with the sole invention of time-sharing, these researchers and their teams played crucial roles in the conceptualization and implementation of time-sharing systems. The idea quickly spread to other institutions and became a fundamental concept in the evolution of computing. Dynamicity which is the underlying principle in this time sharing concept along with the law of evolution of Transition to a Micro-Level suggest the transition from a rigid to a flexible structure. The time-sharing model reflects the dynamic allocation of computing resources to multiple users, enhancing efficiency and flexibility.
One term you might be familiar with is “food truck” or “mobile food truck.” Food trucks are vehicles equipped with kitchen facilities to cook and sell food, often parked at various locations to serve customers. They have become popular in many cities around the world, offering a wide range of cuisines. Food trucks are mobile and can relocate to different areas, events, or neighborhoods based on demand. Despite their small size, food trucks are equipped with functional kitchens that allow chefs to prepare a variety of dishes. Food trucks often specialize in a specific cuisine or type of food, providing diverse and unique options for customers. Customers can enjoy freshly prepared meals without the need to sit in a traditional restaurant.
Food trucks bring food closer to people who might not have easy access to traditional restaurants, especially in busy urban areas or during events. They offer a variety of food options, allowing customers to choose from different cuisines or styles in one location. Food trucks are known for their quick service, making them a convenient option for individuals with limited time. Food truck meals can often be more affordable than dining in a restaurant, making them accessible to a broader range of customers. Food trucks often showcase culinary innovation, introducing new and unique dishes to the market.
While food trucks have become popular and offer numerous benefits, challenges such as zoning regulations, limited space, and competition can impact their operations. However, they continue to be a popular and dynamic part of the food industry, providing a unique dining experience for customers.
On the other hand, for medical professionals or emergencies, vehicles like ambulances that become appropriate in healthcare industry (making certain patient care facilities available in-transit). Ambulances are specially designed and equipped vehicles used to transport patients, including those in critical conditions, to medical facilities. They are equipped with medical equipment and trained personnel to provide immediate care during transit. In some cases, mobile clinics or medical vans are used to bring healthcare services to specific communities or areas that may have limited access to traditional healthcare facilities. These vans are equipped with basic medical facilities to provide services such as check-ups, vaccinations, and consultations.
Mobile libraries or bookmobiles are vehicles, often vans or buses, that are equipped to serve as libraries on wheels. They bring library services, books, and other materials to areas that may not have easy access to traditional library facilities. Mobile libraries enhance access to library services in rural or underserved areas, bringing books and resources to communities that may not have a nearby library. Bookmobiles engage in community outreach, supporting literacy initiatives and promoting the joy of reading. They may visit schools, community centers, or other gathering places. Despite limited space, bookmobiles carry a diverse collection of books for various age groups and interests. They often include fiction, non-fiction, children’s books, and more. Some bookmobiles host events, storytelling sessions, and educational programs, creating a dynamic and interactive experience for users.
Mobile libraries can adapt to changing needs and locations. They can serve different communities on scheduled routes, making them versatile in reaching a wide audience. By bringing books directly to people, bookmobiles play a role in promoting literacy and a love for reading, especially among children. Bookmobiles have limited space compared to traditional libraries, impacting the number of books they can carry. Managing and maintaining a mobile library can involve operational costs, including fuel, maintenance, and staff. Mobile libraries are a valuable resource, particularly in areas where establishing a permanent library might be challenging. They contribute to fostering a reading culture and providing educational opportunities to diverse communities
Using multiple screens aligns with many principles, primarily Dynamicity, Segmentation, Another Dimension, and Extaction, to address contradictions related to limited space, multitasking needs, and task-specific efficiency. Multiple screens provide additional workspace, allowing users to spread out applications, documents, and tasks across different screens simultaneously. Multiplication (increasing the number of screens) helps resolve the contradiction between having limited screen space and the need to view and manage multiple applications or documents. Users can work on multiple tasks simultaneously without constantly switching between applications. Each screen can host a different task or application. Dynamicity, and/or Local Quality (adding different screens for same or different tasks) helps resolve the contradiction between the desire to multitask efficiently and the limitations of a single-screen setup.Larger viewing area improves visibility and reduces the need for scrolling. Users can compare information side by side without overlapping. Segmentation, Extraction, Local Quality and Dynamicity work together to address the contradiction between limited screen real estate and the need for clear visibility of multiple items. Users can arrange screens based on their workflow, creating a seamless and organized working environment. Different screens can be dedicated to specific tasks, such as monitoring data, communication, or reference materials, contributing to task-specific efficiency.
The adoption of a grading system instead of a binary pass or fail approach aligns with this principle as wellas with the law of tansition to a micro-level. This principle emphasizes the transition from rigid, fixed structures to more flexible or dynamic ones. In the context of education, the shift to a grading system introduces a more nuanced and flexible approach to evaluating students’ performance, allowing for a finer granularity in assessing their achievements and progress.
Also, the situation where students are no longer assigned to a fixed grade and have a flexible curriculum based on their abilities and interests, aligns with the educational concept of “Differentiated Instruction” or “Individualized Learning.” This approach reflects the recognition that individuals have unique learning styles, abilities, and interests. Differentiated Instruction involves tailoring teaching methods, content, and assessment to address the diverse needs of students. It allows students to progress at their own pace, explore areas of personal interest, and receive support where needed. This approach aims to enhance student engagement and achievement by recognizing and accommodating individual differences. In the context of inventive principles, the flexibility and adaptability inherent in Differentiated Instruction may resonate with principles related to system dynamization or the transition from rigid to flexible structures or resonance. While not a direct match to a specific TRIZ principle, the educational approach described reflects a dynamic and adaptive system that responds to the diverse needs of learners.
Also, the situation where students are no longer assigned to a fixed grade and have a flexible curriculum based on their abilities and interests, aligns with the educational concept of “Differentiated Instruction” or “Individualized Learning.” This approach reflects the recognition that individuals have unique learning styles, abilities, and interests. Differentiated Instruction involves tailoring teaching methods, content, and assessment to address the diverse needs of students. It allows students to progress at their own pace, explore areas of personal interest, and receive support where needed. This approach aims to enhance student engagement and achievement by recognizing and accommodating individual differences. In the context of inventive principles, the flexibility and adaptability inherent in Differentiated Instruction may resonate with principles related to system dynamization or the transition from rigid to flexible structures or resonance. While not a direct match to a specific TRIZ principle, the educational approach described reflects a dynamic and adaptive system that responds to the diverse needs of learners.
This principle aligns closely with the concept of overcoming the continued influence effect in technical systems. By applying this principle, technical systems can proactively address the continued influence effect and maintain relevance, effectiveness, and competitiveness in dynamic environments. It emphasizes the importance of introducing changes or variations into a system over time to prevent stagnation or the persistence of outdated practices. It involves continuously evolving and adapting the system’s structure, processes, or components to remain responsive to changing conditions, requirements, and opportunities. In the context of addressing the continued influence effect in technical systems, it supports or encourages proactive measures to introduce dynamic changes that counteract the tendency to rely on outdated information or practices. This may include strategies such as: Regularly updating and refreshing system components, technologies, or methodologies to incorporate the latest advancements and knowledge. Implementing mechanisms for ongoing monitoring, evaluation, and feedback to identify and correct misinformation or outdated beliefs in technical decision-making processes. Promoting a culture of innovation, experimentation, and continuous improvement to foster agility and adaptability in responding to changing circumstances. Encouraging interdisciplinary collaboration and cross-functional communication to facilitate the exchange of new ideas, insights, and best practices across different domains. Providing training and education programs to equip individuals with the skills and knowledge needed to discern and address the influence of misinformation in technical systems effectively.
Naive realism is a cognitive bias or theory of perception that describes the tendency of individuals to believe that their perception of reality is accurate and objective, and that those who disagree with them must be uninformed, irrational, or biased. In other words, people tend to assume that their own perspective is the only true and valid one, and they often struggle to understand or accept alternative viewpoints. Key features of naive realism include: Belief in Objectivity: Individuals tend to believe that their perceptions accurately represent the objective reality of the world around them. They may assume that others see the world in the same way and that differences in perception are due to ignorance or bias. Lack of Awareness of Bias: Naive realists may be unaware of the biases and cognitive processes that shape their own perceptions and beliefs. They may assume that their judgments are based solely on objective facts, rather than influenced by subjective factors. Difficulty in Understanding Others: Naive realists may struggle to understand or empathize with individuals who hold different beliefs or perspectives. They may attribute differences in opinion to moral or intellectual deficiencies in others, rather than recognizing the role of differing experiences, values, and interpretations. Tendency to Polarization: Naive realism can contribute to polarization and conflict, as individuals may become entrenched in their own viewpoints and dismissive of alternative perspectives. This can hinder constructive dialogue and cooperation.
To overcome the limitations of naive realism, individuals can: Cultivate awareness of their own biases and the cognitive processes that influence perception and judgment. Practice empathy and actively seek to understand the perspectives of others, even if they disagree with them. Engage in open-minded dialogue and consider alternative viewpoints without immediately dismissing them. Recognize the complexity of reality and acknowledge that multiple perspectives may coexist, each offering valuable insights. By acknowledging the limitations of naive realism and adopting more nuanced approaches to perception and understanding, individuals can enhance their capacity for empathy, critical thinking, and constructive engagement with others.
Naive realism can have significant implications for designing systems, particularly those that involve interactions between users with diverse perspectives and backgrounds. Here are several ways in which naive realism can influence system design: User Interfaces and Experience: Naive realism can influence the design of user interfaces by leading designers to assume that all users perceive and interact with the system in the same way. Designers may overlook the diverse range of user preferences, needs, and cognitive abilities, resulting in interfaces that are not inclusive or accessible to all users. Personalization and Customization: Naive realism may lead designers to prioritize their own preferences and assumptions about user needs when designing personalized or customizable features. This can result in systems that offer limited flexibility or fail to adequately accommodate the diverse preferences and requirements of users. Algorithmic Bias: Naive realism can contribute to algorithmic bias in systems that rely on automated decision-making processes. Designers may assume that algorithms are objective and neutral, overlooking the potential for biases to be embedded in the data or design of the algorithms themselves. This can result in systems that produce biased outcomes, particularly for marginalized or underrepresented groups. Feedback and Iterative Design: Naive realism may hinder the effectiveness of feedback mechanisms and iterative design processes by leading designers to dismiss user feedback that contradicts their own perceptions or assumptions about the system. Designers may be less inclined to consider alternative viewpoints or iterate on designs based on user input, resulting in systems that fail to address user needs or preferences.
To mitigate the impact of naive realism on system design, designers can: Adopt user-centered design principles that prioritize understanding user needs, preferences, and behaviors through research and user testing. Foster a culture of empathy, open-mindedness, and collaboration within design teams to encourage consideration of diverse perspectives and feedback. Incorporate mechanisms for inclusive design, such as user personas, accessibility guidelines, and usability testing with diverse user groups. Implement transparency and accountability measures to identify and address biases in algorithms and decision-making processes. By acknowledging and addressing the influence of naive realism on system design, designers can create more inclusive, effective, and user-centered systems that better serve the needs of all users.
Zero-Sum Bias: The zero-sum bias is the tendency to perceive situations as zero-sum games, where one person’s gain is perceived as another person’s loss. In designing a technical system, this bias might lead to a focus on competition rather than collaboration, resulting in adversarial relationships between different stakeholders or teams. When solving technical problems, individuals might prioritize their own interests over collective goals, leading to conflicts or suboptimal solutions that do not benefit all parties involved.
11: Tension, Pressure: [‘5: Area of the moving object’, ‘6: Area of the non-moving object’, ’12: Shape’]
12: Shape: [‘2: Mass of the non-moving object’, ‘7: Volume of the moving object’, ‘9: Speed’, ’11: Tension, Pressure’, ’18: Brightness, Visibility’, ’33: Convenience of use’, ’35: Adaptability’, ’37: Complexity of control and measurement’, ’38: Level of automation’]
13: Stability of the object: [‘3: Length of the moving object’, ‘9: Speed’, ’14: Strength’, ’26: Amount of substance’]
14: Strength: [‘1: Mass of the moving object’, ‘3: Length of the moving object’, ‘4: Length of the non-moving object’, ‘7: Volume of the moving object’, ‘8: Volume of the non-moving object’, ’31: Harmful internal factors’, ’35: Adaptability’, ’37: Complexity of control and measurement’, ’38: Level of automation’]
15: Action time of the moving object: [’30: Harmful external factors’, ’36: Complexity of the structure’]
17:Temperature: [‘3: Length of the moving object’, ‘4: Length of the non-moving object’, ’19: Energy consumption of the moving object’, ’39: Productivity’]
18: Brightness, Visibility: [’20: Energy consumption of the non-moving object’, ’28: Accuracy of measurement’, ’30: Harmful external factors’, ’34: Convenience of repair’, ’35: Adaptability’, ’37: Complexity of control and measurement’]
19: Energy consumption of the moving object: [‘5: Area of the moving object’, ’18: Brightness, Visibility’, ’22: Energy loss’, ’34: Convenience of repair’, ’35: Adaptability’]
21: Power: [‘9: Speed’, ’13: Stability of the object’, ’28: Accuracy of measurement’]
22: Energy loss: [‘1: Mass of the moving object’, ‘5: Area of the moving object’, ’18: Brightness, Visibility’, ’37: Complexity of control and measurement’]
23: Material loss: [’10: Force’, ’25: Time loss’, ’32: Convenience of manufacturing’, ’35: Adaptability’]
24: Information loss: [’39: Productivity’]
25: Time loss: [‘3: Length of the moving object’]
26: Amount of substance: [‘5: Area of the moving object’, ‘7: Volume of the moving object’, ’13: Stability of the object’, ’35: Adaptability’]
27: Reliability: [‘3: Length of the moving object’, ‘4: Length of the non-moving object’]
30: Harmful external factors: [’15: Action time of the moving object’]
31: Harmful internal factors: [‘1: Mass of the moving object’, ‘3: Length of the moving object’, ’14: Strength’, ’15: Action time of the moving object’]
32: Convenience of manufacturing: [‘1: Mass of the moving object’, ‘4: Length of the non-moving object’, ’23: Material loss’, ’35: Adaptability’]
33: Convenience of use: [‘1: Mass of the moving object’, ‘6: Area of the non-moving object’, ‘7: Volume of the moving object’, ’12: Shape’, ’35: Adaptability’, ’39: Productivity’]
34: Convenience of repair: [‘5: Area of the moving object’, ’18: Brightness, Visibility’, ’19: Energy consumption of the moving object’, ’21: Power’, ’22: Energy loss’, ’33: Convenience of use’]
35: Adaptability: [‘1: Mass of the moving object’, ‘2: Mass of the non-moving object’, ‘6: Area of the non-moving object’, ‘7: Volume of the moving object’, ’10: Force’, ’12: Shape’, ’22: Energy loss’, ’23: Material loss’, ’26: Amount of substance’, ’33: Convenience of use’, ’36: Complexity of the structure’]
36: Complexity of the structure: [’12: Shape’, ’15: Action time of the moving object’, ’35: Adaptability’, ’37: Complexity of control and measurement’, ’38: Level of automation’]
37: Complexity of control and measurement: [’14: Strength’, ’22: Energy loss’, ’35: Adaptability’, ’36: Complexity of the structure’]
38: Level of automation: [’12: Shape’, ’36: Complexity of the structure’]
39: Productivity: [‘2: Mass of the non-moving object’, ’10: Force’, ’24: Information loss’]
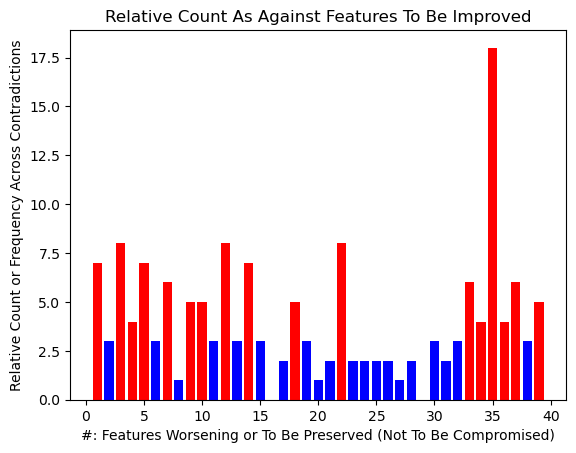
1/3 1/9 1/35 2/21 2/22 2/24 2/35 2/37 2/39 3/1 3/5 3/13 3/17 3/25 3/30 3/31 3/33 3/35 4/12 4/14 4/27 4/32 5/3 5/11 5/14 5/17 5/18 5/22 5/33 5/34 5/35 6/11 6/35 6/39 7/10 7/12 7/14 7/22 7/33 7/35 8/14 9/10 9/12 9/19 9/35 10/5 10/7 10/9 10/22 10/32 10/34 10/35 11/5 11/6 11/12 12/2 12/7 12/9 12/11 12/18 12/33 12/35 12/37 12/38 13/3 13/9 13/14 13/26 14/1 14/3 14/4 14/7 14/8 14/31 14/35 14/37 14/38 15/30 15/36 17/3 17/4 17/19 17/39 18/20 18/28 18/30 18/34 18/35 18/37 19/5 19/18 19/22 19/34 19/35 21/9 21/13 21/28 22/1 22/5 22/18 22/37 23/10 23/25 23/32 23/35 24/39 25/3 26/5 26/7 26/13 26/35 27/3 27/4 30/15 31/1 31/3 31/14 31/15 32/1 32/4 32/23 32/35 33/1 33/6 33/7 33/12 33/35 33/39 34/5 34/18 34/19 34/21 34/22 34/33 35/1 35/2 35/6 35/7 35/10 35/12 35/22 35/23 35/26 35/33 35/36 36/12 36/15 36/35 36/37 36/38 37/14 37/22 37/35 37/36 38/12 38/36 39/2 39/10 39/24
EXAMPLE: One example of applying the dynamicity principle to increase adaptability without increasing complexity can be seen in a heating, ventilation, and air conditioning (HVAC) system with dynamic climate control.
Contradition (35/22, 35/33 and 35/36): Consider an HVAC system in a building that needs to adapt to varying occupancy levels and changing weather conditions while avoiding unnecessary energy consumption.
Solution: The dynamicity principle is applied to create a HVAC system that dynamically adapts to various factors while maintaining a manageable level of complexity. The system’s adaptability ensures efficient and comfortable operation without unnecessary intricacies. Implement occupancy sensors that dynamically adjust temperature settings based on the number of people in a room. The system remains straightforward, and the addition of sensors doesn’t significantly increase complexity. Integrate weather forecasting data to anticipate temperature changes and adjust HVAC settings preemptively. The integration is designed to be seamless and doesn’t introduce unnecessary complexity to the core HVAC system. Adjust temperature settings based on the time of day to optimize energy usage. The system is designed to handle time-based adjustments without a substantial increase in complexity. Implement machine learning algorithms to learn and adapt to individual user preferences over time. While machine learning is introduced, the system is designed to handle the learning process without making the overall HVAC system overly complex. The dynamic HVAC system adapts to specific conditions, optimizing energy usage without compromising comfort. Individualized adjustments based on occupancy and user preferences enhance user comfort without manual interventions. Anticipatory adjustments based on weather forecasts contribute to reducing the environmental impact of the HVAC system. The system can scale to handle additional features, sensors, or adaptive mechanisms without significantly increasing its inherent complexity.